Best Practices: Extending the Life of Lithium-Ion Batteries
Key Takeaways
- High current and voltage exposure negatively impacts lithium-ion batteries’ performance, safety, and lifespan.
- Avoid fully draining lithium-ion batteries; partial charging is better for longevity.
- Maintain charge within the 20%-80% range for optimal performance and reduced wear.
- Battery temperature is crucial; keep it within the ideal range for extended life.
- Managing charge and discharge rates can help balance performance demands with longevity.
Introduction
Lithium-ion batteries are pivotal across a wide swath of industries, powering everything from consumer electronics to electric vehicles and renewable energy storage systems. Their widespread use has amplified the need for strategies that extend their lifespan, both to reduce environmental impact and save on hefty replacement costs. Understanding factors that contribute to battery degradation—such as environmental conditions, state of charge (SoC), charging/discharging rates, and current exposure—enables users to mitigate wear and maximize battery life.
Anatomy of a Lithium-Ion Battery
A lithium-ion battery cell consists of four primary components:
Anode (Negative Electrode): Holds lithium ions during discharge.
Cathode (Positive Electrode): Stores lithium ions when the battery is charged.
Separator: Prevents direct contact between the anode and cathode, allowing ions to pass between them during charging and discharging.
- Electrolyte: A medium that facilitates ion movement between the electrodes.
During discharge, lithium ions flow from the anode to the cathode through the electrolyte, producing electrical energy. This process reverses during charging as ions move from the cathode back to the anode. While lithium-ion cells power small devices like smartphones, combining them into modules creates larger battery packs suitable for electric vehicles, factories, and energy storage systems. Despite their versatility and energy density, lithium-ion batteries inevitably degrade with each cycle, gradually reducing their capacity and performance.
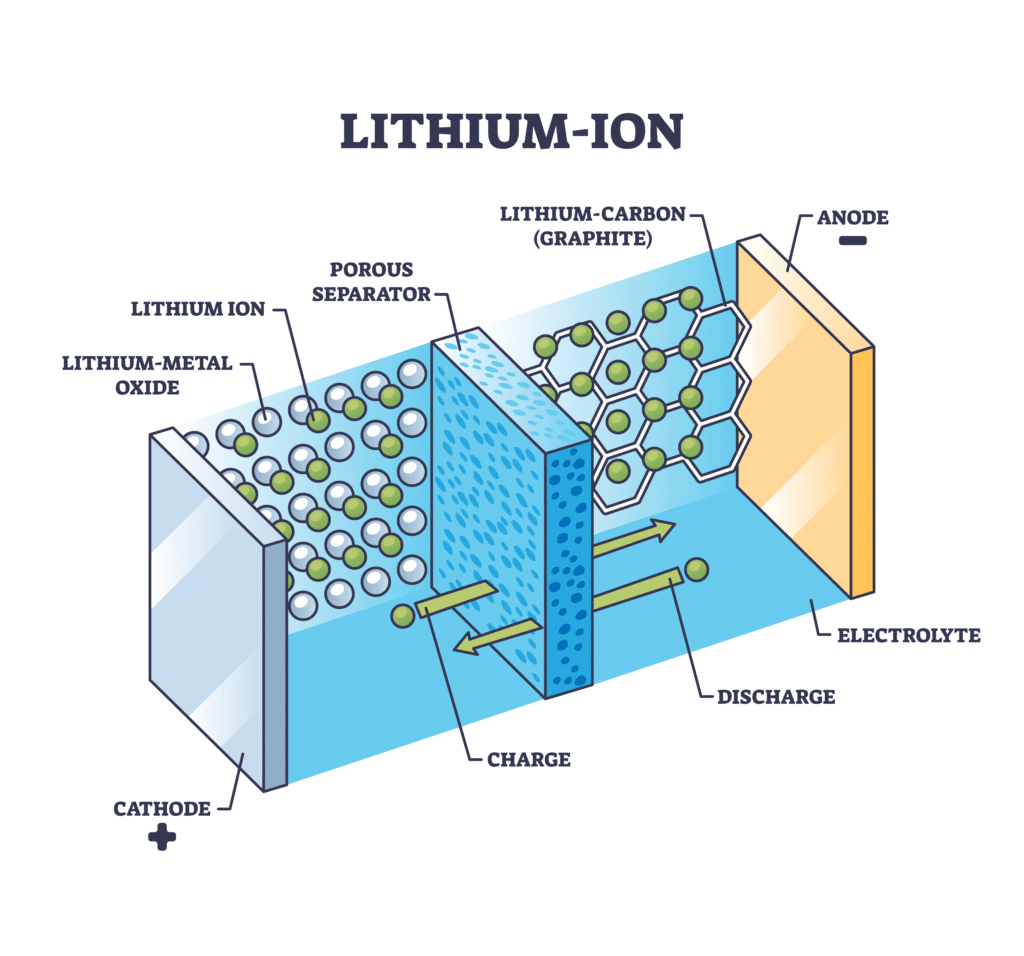
Exposure to High Current
High current exposure—whether during charging or discharging—poses risks that accelerate battery wear, including:
- Excessive Heat Generation: High currents amplify heat, and without proper temperature control, this can push the battery beyond safe operating conditions.
- Chemical Degradation: High currents cause reactions in the electrolyte, reducing its efficacy and contributing to unwanted byproducts that increase internal resistance.
- Mechanical Stress: Rapid ion movement causes expansion and contraction within the electrodes. Over time, this physical stress creates micro-cracks that degrade performance and reduce capacity.
Limiting high current exposure, especially during charging, helps extend battery life. A combination of a BMS, current limiters, and temperature management systems can control currents within optimum limits, reducing risks and preserving performance. According to industry research, “high currents can affect the cycle life of lithium-ion batteries…exposing them to mechanical stress, heat stress, and parasitic side reactions that degrade the battery.”⁴
Exposure to Transient Voltage
Similarly, transient voltage (sudden surges in voltage) can have harmful effects on lithium-ion batteries, impacting their performance, safety, and lifespan. These voltage spikes, if unmitigated, can lead to chemical degradation, thermal runaway, capacity loss, and even safety hazards.
- Chemical Degradation: Transient voltage can cause brief periods of overcharging in the battery, which accelerates unwanted chemical reactions in the electrolyte and electrode materials. This can result in the formation of lithium plating on the anode, reducing the battery’s overall capacity and efficiency over time. Repeated transient events cause further chemical imbalances, which may lead to internal short circuits within the battery.
- Thermal Runaway: High transient voltages can generate excess heat within the battery. This poses a risk of thermal runaway, a dangerous process where heat from exothermic reactions causes a domino effect. If thermal runaway progresses unchecked, it can lead to the venting of harmful gasses, fires, or even explosions, especially in applications requiring high power demands.
- Capacity Loss: Frequent transient voltages can permanently reduce the battery’s energy storage capability by damaging the electrolyte and electrode structures, leading to faster capacity degradation. Even short, infrequent transients, if repeated over time, can decrease the battery’s efficiency and shorten its lifespan.
- Safety Risks and Reduced Lifespan: Unmanaged transients can compromise the structural integrity of the battery, increasing the risk of swelling, venting, and internal shorts. This poses a safety hazard and can reduce the operational life of the battery, particularly when the protective circuitry (BMS) is unable to handle repeated transient events.
Proper mitigation of transient voltages is essential to protect lithium-ion batteries, enhance their safety, and extend their useful life. While a BMS provides some protection, additional methods can further reduce the effects of transient voltage. Transient voltage surge suppressors should be placed close to the battery itself, react faster than a nano-second, and be appropriately sized to the immunity level of the battery.
Environmental Conditions
Environmental conditions significantly affect lithium-ion battery lifespan. Key factors include:
1. Temperature Range: The ideal operating temperature for most lithium-ion batteries is between 50°F and 80°F (10°C – 27°C). Operating outside this range can accelerate degradation:
High Temperatures: Heat accelerates chemical reactions within the battery, causing irreversible damage and increasing the risk of thermal runaway, where excessive heat causes the battery to overheat further, potentially leading to fires or explosions.
Low Temperatures: Cold increases internal resistance, making it harder to charge the battery and reducing its effective capacity during discharge.
These effects are particularly pronounced during charging, as the battery’s chemical stability is most vulnerable at this time. High temperatures increase stress on the electrolyte and other components, while low temperatures slow the movement of lithium ions, both of which can shorten battery life.
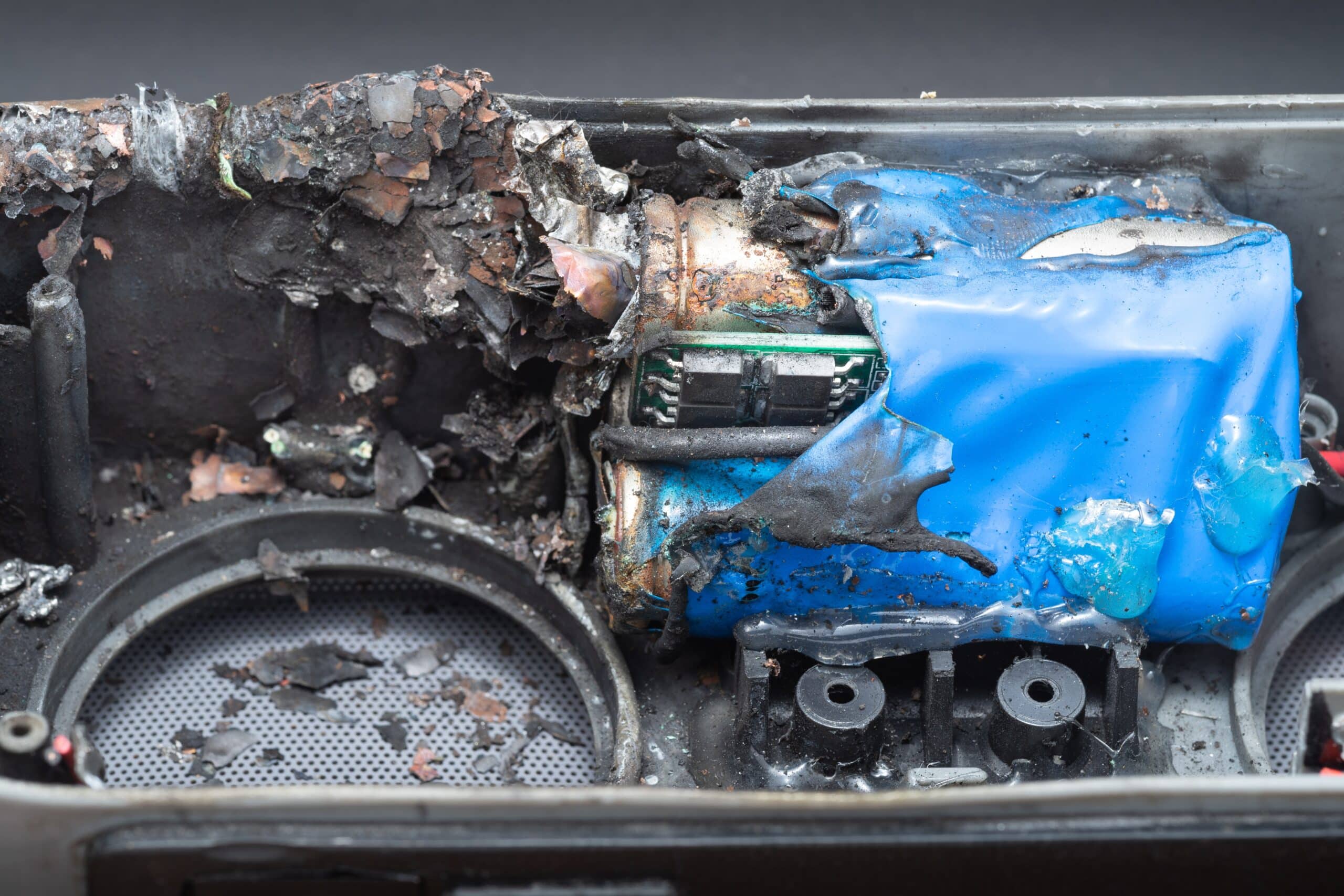
2. Humidity and Ventilation: Lithium-ion batteries also benefit from proper airflow and humidity control. During operation, batteries generate heat due to internal resistance and chemical reactions. Inadequate ventilation can trap this heat, forcing the battery to operate at higher temperatures than its environment, which leads to faster degradation. High humidity or moisture exposure can compromise the electrolyte, the component most sensitive to such conditions, resulting in chemical instability and potential leakage.
State of Charge (SoC) Management
Since their commercial debut in 1991, lithium-ion batteries have proven more reliable and longer-lasting than alternatives largely due to their lack of “memory effect”—a phenomenon in which certain battery types lose capacity if not fully discharged before recharging. Instead, lithium-ion batteries degrade over a cycle count basis, where each complete cycle (from full charge to full discharge) causes a small but cumulative loss in capacity.
Research has shown that a 20-80% SoC window is ideal for preserving battery health. At this range:
- Voltage is more stable, placing less stress on battery components.
- When charge drops below 20%, voltage levels decrease rapidly, accelerating wear on the electrodes.
- Above 80%, voltage levels increase slowly, causing added stress on the electrolyte and increasing the risk of lithium plating, a harmful process that reduces available lithium ions and shortens battery life.
Many modern devices use a Battery Management System (BMS) to prevent overcharging and deep discharges. By keeping the battery within optimum SoC levels, these systems reduce cycle wear and extend lifespan.
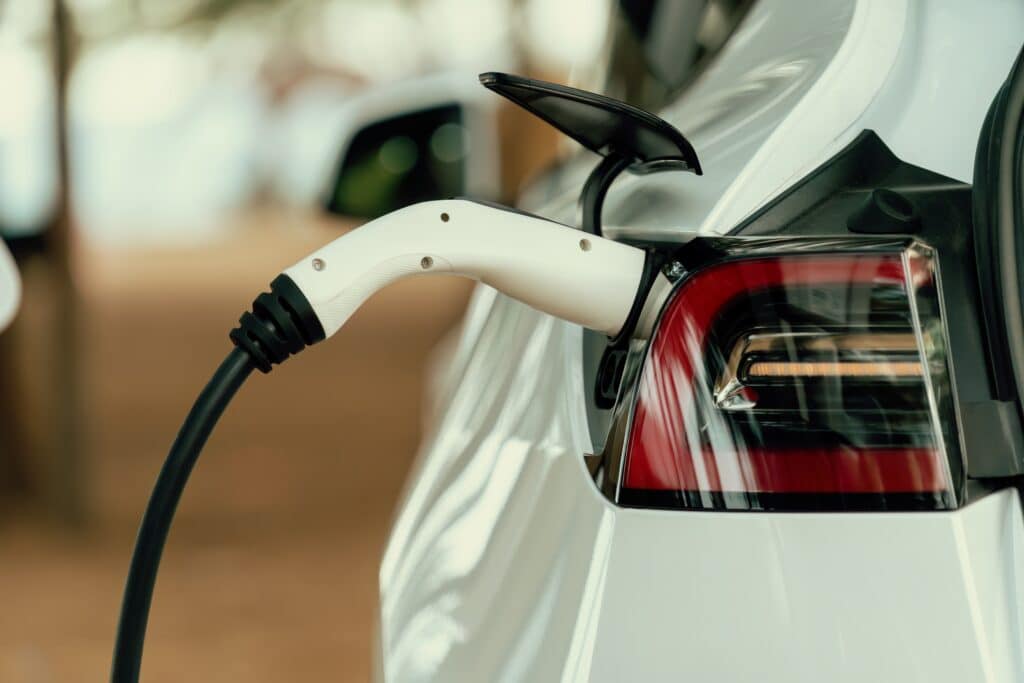
Charging and Discharging Rates
The rate at which lithium-ion batteries are charged or discharged (commonly referred to as C-rate) impacts both efficiency and longevity:
- Normal C-Rates: Typically between 0.5C to 1C for most applications (where 1C means a battery charges fully in one hour), these moderate rates provide a balance between charge speed and battery longevity.
- Fast Charging: Ranging from 1C to 3C in some consumer devices, fast charging significantly reduces charge time but increases degradation due to higher heat generation and chemical stress.
- High Discharge Rates: Applications requiring intense power output, like drones or power tools, often operate at discharge rates between 5C to 15C. Although they deliver power quickly, these high rates increase internal heat and stress, which can shorten overall battery life.
Managing charge and discharge rates can help balance performance demands with longevity. For high-performance devices, limiting frequent high-rate charging/discharging can mitigate wear.
Conclusion
Optimizing lithium-ion battery lifespan requires understanding how environmental conditions, SoC management, charging/discharging rates, and current and transient voltage exposure impact battery health. With careful handling and utilization of modern technology such as BMS, controlled charging infrastructure, and transient voltage mitigation, users can significantly extend the lifespan and reduce safety risks of these essential power source. This results in reduced environmental impacts, lower costs over time, and enhanced safety.
Resources
-
Dauley, G. (2021, August 12). How it works: delving into the anatomy of a battery. PacificGreen.com. Retrieved October 28, 2024, from https://www.pacificgreen.com/articles/how-it-works-delving-anatomy-battery/
-
M. Woody, M. Arbabzadeh, G.M. Lewis, G.A. Keoleian, A. Stefanopoulou. Strategies to limit degradation and maximize Li-ion battery service lifetime – Critical review and guidance for stakeholders. J. Energy Storage. 28 (2020). doi:10.1016/j.est.2020.101231.
-
Erickson, J. (2020, February 17). Tips for extending the lifetime of lithium-ion batteries. University of Michigan News. Retrieved October 28, 2024, from https://news.umich.edu/tips-for-extending-the-lifetime-of-lithium-ion-batteries/
-
Factors influencing longevity of lithium-ion batteries. (2024, May 7). Lohum. Retrieved October 28, 2024, from https://lohum.com/media/blog/factors-influencing-longevity-of-lithium-ion-batteries/#:~:text=Lithium%20Ion%20battery%20life%20varies,and%20regulatory%20and%20safety%20factors
-
Maximizing Battery Efficiency: A guide to cycle life and its impact. (2023, December 5). Bioenno Power. Retrieved October 28, 2024, from https://www.bioennopower.com/blogs/news/understanding-battery-cycle-life-importance#:~:text=One%20of%20the%20most%20effective,unnecessary%20stress%20on%20the%20battery
-
Ghafari, A., Bayat, V., Akbari, S., & Ghasemi Yeklangi, A. (2023). Current and future prospects of Li-ion batteries: A review. In NanoScience Technology (Volume 8; Vol. 8, pp. 24–43). Retrieved October 28, 2024, from https://jnanoscitec.com/wp-content/uploads/2023/07/Volum-8-paper-2.pdf
-
BU-808B: What causes Li-ion to die? (2021, November 3). Battery University. Retrieved October 28, 2024, from https://batteryuniversity.com/article/bu-808b-what-causes-li-ion-to-die#:~:text=To%20achieve%20this%2C%20the%20cells,SEI%20buildup%20on%20the%20anode